The last decade has witnessed dramatic progress in three directions -- lattice quantum chromodynamics (LQCD), nuclear effective field theories (NEFT), and ab initio nuclear-structure methods (AiM) -- all of which aim to derive and describe nuclear physics with fully controlled uncertainties in a manner consistent with QCD. To follow up on the INT's 2013 workshop on "Nuclear reactions from Lattice QCD", which was received well by the community at the time and promoted interactions among different subdiciplines, and given the amount of progress in the years that followed, and the promising prospect of nuclear physics research in connecting to QCD, the INT hosted a longer program in "Nuclear Physics from Lattice QCD" during Spring of 2016. This ten-week program brought together researchers in the three subdiciplines of nuclear physics to present new results, to share recent advances in various methodologies, and most importantly and more extensively, to discuss specific problems and roadblocks that hinder the progress towards achieving the goal of calculating nuclear physics directly from the underlying theory. This mission was accomplished to a high degree, given participants' strong and diverse background, and the extent and depth of discussions that occurred continuously throughout the program. Here, we summarize some of the scientific outcomes of the program along with a short analysis of the program attendance and the contribution of different sub-groups to the program.
After several years of development and continuous effort, LQCD calculations are fulfilling their promise of calculating basic static and dynamical properties of hadrons with controlled uncertainties. The field has reached the point where single-meson and single-baryon properties can be (post)predicted with precision, and multi-meson calculations are becoming advanced enough to study the excited spectrum of QCD and hadronic resonances, as reported in this program. Substantial work is now in progress to study the properties of light nuclei by collaborations in the US and Japan. Several members of these collaborations actively participated in this program to report on recent progress and discuss and resolve disagreements that have persisted over years regarding some of the results. Multinucleon systems are significantly more difficult to calculate than single-baryon and multi-meson states, as they are more complex in terms of their QCD degrees of freedom, demand larger lattice volumes, require higher precision to account for the fine-tuning of the nuclear forces, and perhaps most importantly suffer from a poor signal-to-noise ratio. Additionally, the other direction of research in the field, namely the studies of QCD phase diagram at finite baryon chemical potential with LQCD, is hindered by a severe sign problem. Algorithmic advances of the past have enabled tackling some of these problems, and new advances in moving towards affordable multi-nucleon calculations are in progress. Two of such advances, one in devising a genuine method to generate QCD gauge configurations toward the continuum limit at a low cost, and one in learning interesting lessons from some toy models about a strategy in tackling the sign problem (Lefschetz thimbles), were presented in this program and generated excitement among LQCD researchers and others.
Currently there are four LQCD collaborations who have devoted their major effort to studying multi-baryon physics, with several other groups (e.g., the Mainz group) conducting single projects that involve more than one baryon. These collaborations (NPLQCD, HALQCD, PACS-CS and CalLat) have been producing exciting results in few nucleon (and hyper-nucleon) systems for pion masses in the range from 300 MeV to 800 MeV (NPLQCD collaboration have obtained results beyond the binding energies and scattering parameters and have studied the magnetic properties of nuclei and hyper-nuclei, as well as a reaction cross section in the two-nucleon system from QCD, as presented in this program). Since the scattering amplitude of Minkowski infinite-volume physics cannot be directly extracted from the Euclidean LQCD correlation functions in a finite volume, one needs to use the Lüscher formalism to extract the scattering amplitude, and consequently the binding energies, from the energy eigenvalues of QCD in a finite volume. This is a well-established method which is taken by most of the collaborations in this fields, leading to consistent results for the same quantities. Formal developments to generalize this approach to more than two hadrons have been going on for several years, and the most recent progress, along with an alternative approach in studying multi-coupled channel systems, were reported in the program. The HALQCD collaboration, on the other hand, have developed and employed a different approach that extracts the baryon-baryon interaction potential directly from the LQCD "wavefunctions". The results obtained within this approach seem to disagree with the evolution of the nuclear binding energies with the pion mass as claimed by other collaborations. Critical views were discussed in this program regarding both approaches. While HALQCD approach was criticized for being model-dependent (due to dependence on lattice interpolators) away from the energy eigenvalues of the volume, the Lüscher's approach was questioned to be problematic due to inaccurate plateau identifications in the long-time behavior of multi-nucleon correlators with significant noise. The limitations and caveats of different approaches, as well as possible future tests, were the subject of heated debates during the program. This gave the participants outside the LQCD field a great opportunity to get familiarized with some of the technologies and concepts prevalent in this field and therefore the ability to evaluate different claims on their own. There was a sense that the interactions among the different groups is going to initiate tests and benchmarks, and will eventually clarify the situation.
At heavier light-quark masses, the formation of quark-antiquark pairs is suppressed, the computational resources required to generate LQCD configurations are reduced, and the signal-to-noise ratio in multinucleon correlation function improves. Therefore, present LQCD calculations of nuclei are performed at heavy up and down quark masses, which result in unphysical values for nucleonic quantities, but serve as a proof of principle that the physical-point calculations are indeed possible once the requisite computational resources are provided. The LQCD results for multinucleon systems at unphysical pion masses, nonetheless, have lead to an exciting direction of research in the field that mimics the (dream and planned) situation in future in which the physical-point LQCD calculations of light nuclei will serve to constrain the input to effective field theories of few-nucleon systems and consequently the ab initio many-body calculations of medium-mass to heavy nuclei. Several of the talks in the program were dedicated to NEFT analyses of the available LQCD data at heavy light-quark masses. These analyses serve as a consistency check of the LQCD calculations and provide an efficient approach to extrapolate the LQCD data to larger systems and different pion masses. Indeed, the results presented in the program show a remarkable consistency between the LQCD results for light nuclei and NEFT calculations of the bound A≤4 systems. There seems to exist some inconsistencies between low-energy theorems, as presented in this program, and the scattering results from LQCD calculations at mπ≈ 500 MeV, a situation that should be resolved once more refined LQCD calculation at this pion mass are available. First applications of potentials extracted from LQCD data to study the structure of the nuclear landscape were presented. Conspicuously absent was the discussion of the appropriate theoretical framework for extrapolating LQCD results obtained at unphysical quark masses to the physical point. As was emphasized throughout the program, an ultimate goal of the LQCD program is to provide information about three and four neutron forces, and that of hyper-nuclear forces from QCD. Given that the application of any generalizations of the Lüscher's direct method to few body systems is complicated and most likely not practical in near future, an alternative method is a direct matching between LQCD energy eigenvalues in a given finite volume and the eigenvalues evaluated in the same volume with ab initio methods to tune the interactions. Several preliminary efforts have already started in this direction, as presented in this program, and more investigations and coordination were planned between experts in both communities.
The combination of advanced many-body methods, softening interactions using the similarity renormalization group (SRG), and advanced computers have led to substantial progress in precision nuclear-structure calculations of medium-size nuclei. Comparing different theoretical and computational approaches, a remarkable agreement was found between methods such as the no-core shell model, in-medium SRG, Coupled-Cluster, Self-Consistent Green's Function, and lattice EFT, in calculating the oxygen isotopic chain. Ab initio calculations of Ca (A≈ 50) and Ni (A≈ 80) isotopes were also presented in this program, and compared to available experimental data. A remarkable progress in this field, as was noticed clearly in this INT program, is the improvement in error estimation in nuclear many-body calculations, and a strong consensus that has been developed among the researchers in this area, demanding to deal better with uncertainties of the calculations. Propagation of uncertainties from the interaction to the nuclear many-body problem is on the horizon, with several attempts reported in this program, while quantifying systematic uncertainties associated with truncations in the ab initio methods remain a challenge. The remarkable progress in the ab initio methods, in particular their ability to calculate properties other than energy spectrum, has revealed some inconsistencies in the current approaches that may be hinting to fundamental issues such as renormalizability, range of convergence, and perturbative vs. iterative realizations, etc. Some of the questions raised during the program include: How should we address the problem of fine-tuned interactions? How may one rigorously control the regulator dependencies for different systems? Do the inconsistencies point to the emergence of a new in-medium scale and so we need to explore new power counting schemes, or is it the insufficiency of the order of the existing EFT potentials and their poor constraints that render them inaccurate at heavier systems? Do we need to revisit the NEFT on a deeper level to go to large nucleon number and abandon nucleonic degrees of freedom (at least for bulk properties)? New strategies, such as that proposed and employed by the Argonne group among others to incorporate the saturation in nuclei as a constraint in fitting the interactions to be able to accurately describe heavier isotopes' bindings and charge radii, or the observation by the LEFT collaboration regarding the need to additionally tune internucleon interactions to alpha-alpha scattering to be able to correctly describe larger clusters of alpha particles, were reported in this program and were met with critical viewpoints and extensive discussion. Addressing these types of questions will surely need a unified effort by the three subcommunities to propose and carry out tests and benchmarks that may shed some light on the confusing situation. This program certainly provided one such intense environment that will hopefully lead to progress.
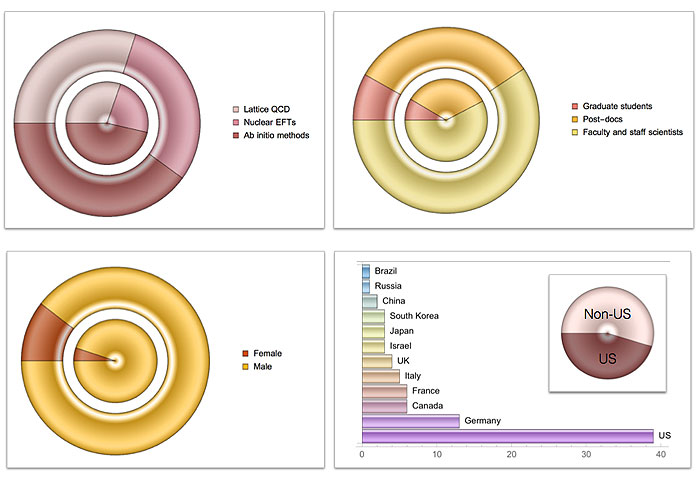
Fig. 2: The breakdown of the INT program participation by expertise, academic rank, gender and country. The outer ring charts represent the breakdown of the full participants while the inner pie charts represent the breakdown of the speakers.
There were a total of 86 official participants in this INT program, with about 30% coming from LQCD, 30% from NEFT and 40% from the AiM communities, as shown in the upper left panel of Fig. 2. As is indicated in the inner chart of the plot, this resulted in more talks on the ab initio methods than other topics. At the same time, an effort was made to create a balance in discussions if it was appropriate given the mixture of the participants each week. This program was a successful example of the positive dynamics generated by a large number of junior researchers in the meeting (28 postdoctoral researchers as well as 7 graduate students), who brought valuable hands-on experience and detail-oriented view to the discussions and at the same time benefited from the diversity and breadth of the viewpoints presented by senior participants. The academic-rank breakdown of the participants is depicted in the upper right panel of Fig. 2, with inner chart indicating the breakdown of the number of talks given by each sub-group. We encouraged and supported applications by female scientists and had the opportunity to host 9 female researchers from the US and abroad in this program. As is plotted in the lower left panel of Fig. 2, the fraction of female participants to the male participants (and consequently that of the number of speakers) remains low in our program, which is a reflection of the low number of female theorists in the field. However, given that a large number of our female participants were young researchers in their early careers, we anticipate a fortunate growth in women's representation in the field in the upcoming years. Last but not least, we had a multi-national program with researchers participating from 12 different countries. As is shown in the lower right panel of Fig. 2, nearly 55% of the participants came from institutions that are based outside the US.
In summary, given all the activities in the three subfields of LQCD, NLEFT and AiM and the level of excitement in the community to reach the goals in this field, we believe this INT program was held at exactly the right time, and was met with success in unifying some of the diverse thoughts and ideas in the community regarding the program of bridging between QCD and nuclear physics. The field is quickly moving forward, has identified the problems, has reached a general consensus in controlling the uncertainties in various stages of a nuclear many-body calculation. Most importantly, it acknowledges the need for precise lattice QCD calculations of several key quantities, and will be providing feedback to such calculations along the way.