 |
Core-collapse supernovae are among the most energetic explosions in the
Universe. As such they play a major role in many astrophysical phenomena,
including galactic dynamics, nucleosynthesis, and neutron star and black
hole formation. Furthermore, the combination of extreme energetics and
densities make these explosions fascinating nuclear and particle physics
laboratories. Hence, a solid theoretical framework for the explosion
mechanism will greatly illuminate solutions to some of the most
important questions in astrophysics and fundamental physics.
The purpose of this program was to advance our understanding
of the explosion mechanism by fostering collaborations among the
theorists, observers, and nuclear and particle physicists. After
many years of intense research, SN theorists are starting to converge
on viable solutions to the explosion mechanism. At the same time,
electromagnetic observations are beginning to probe closer to the
mechanism, and neutrino and gravitational wave dectectors are
coming on line that can probe the mechanism directly. These
multi-messenger observations promise to greatly constrain the
theory. The time is ripe for these communities to share the
latest results, define the next questions in SN research, and
begin to constrain the physics of core-collapse supernovae.
With this goal in mind experts from a wide range of fields
came together for the 5-week program that was organized around
two topical workshops on
"Nuclear and Neutrino Physics in Stellar Core Collapse" and
"Probing the Supernova Mechanism by Observations". The areas
of research represented included
supernova and remnant observations, progenitor and explosion
modeling, nuclear astrophysics of matter under extreme conditions,
neutrino theory and detection, and gravitational-wave astrophysics.
Supernova modeling is presently facing the grand computational
challenge of carrying the simulations to the third dimension.
These efforts were already rewarded with the first successful
explosion models in 3D, although the computations still used
modest numerical resolution and considerable simplifications in
the neutrino treatment, which fall behind the most sophisticated
existing 2D models. As in 2D simulations, the explosions are
triggered by neutrino-energy deposition behind the supernova shock
and are supported by violent nonradial mass motions, which stretch
the timescale that matter is exposed to neutrino heating.
The nature of these nonradial mass motions and the underlying
hydrodynamical instability --- convection and buoyancy or the
so-called standing accretion shock instability (SASI) --- are still a
matter of vivid discussion. It is also unclear whether large-scale
asymmetries (with strong dipolar and quadrupolar components of
the flow pattern) or turbulent energy redistribution are mainly
responsible for differences between models in one, two, and three
dimensions.
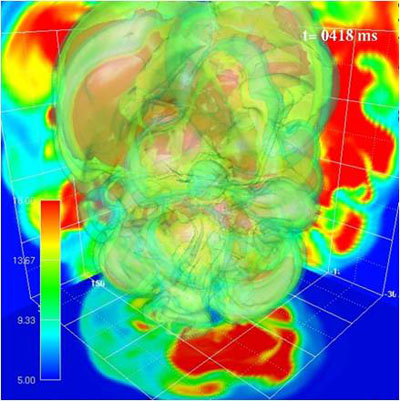
Figures 1a and 1b:
Two snapshots of a three-dimensional
explosion model of an 11.2 solar-mass star at about 350 and 420
milliseconds after core bounce. The transparent surfaces
correspond to different entropy levels and the projections
visualize the entropy distribution by color coding. Large
asymmetries, which have grown from small random perturbations,
are generically connected to the onset of the explosion in
2D and 3D simulations. (Courtesy of Kei Kotake)
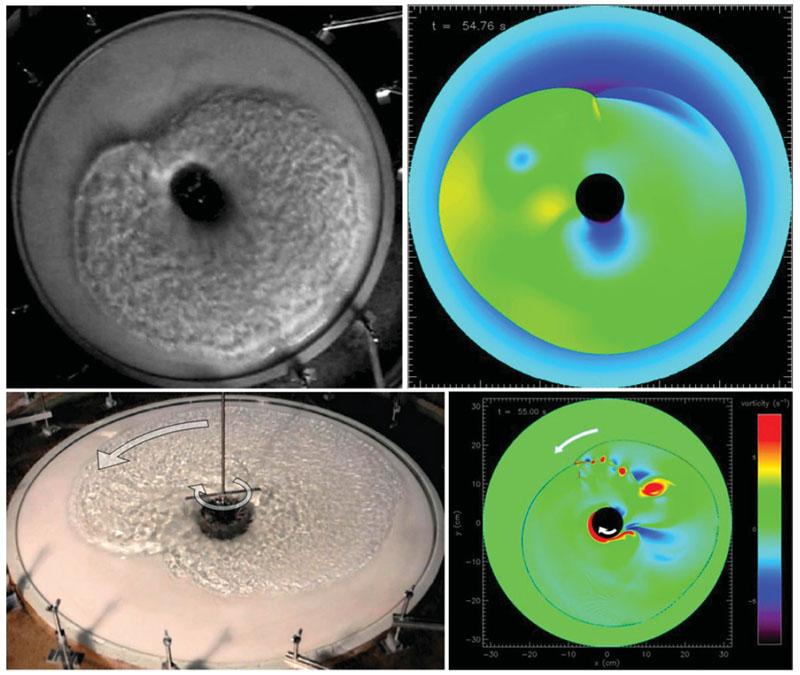
Figure 2:
Shallow-water analogue of the standing accretion shock instability
(SASI). The radial water flow towards a central drain pipe and
a hydraulic jump corresponds to the accretion flow and accretion
shock in the supernova core. A spiral-mode pattern with characteristic,
rotating triple point and angular momentum separation
can spontaneously develop even without initial rotation
in this experimental "SWASI" setup (left images). Hydrodynamical
simulations reproduce the experimental findings (right panels).
(T. Foglizzo et al., Phys. Rev. Lett. 108, 051103 (2012);
copyright: American Physical Society)
Progress on the modeling front is counterparted by a growing
pool of observational data. Supernovae whose progenitors can be
identified on archival images help to constrain the masses and
properties of the corresponding stars. Oxygen lines with multiple
peaks as well as radiation polarization yield evidence of
time dependent explosion asymmetries and suggest a wide range of
deformation from globally anisotropic supernovae to cases with
"clumpy" local inhomogeneities. X-ray, optical, and radio
"tomography" of young supernova remnants begins to reveal features
that can be traced back to the earliest moments of the blast. In
particular the systematic observational dissection of the Cassiopeia A
remnant has revealed a wealth of ring-like structures, which seem to
originate from the bubbles and Rayleigh-Taylor mushrooms
found to carry heavy elements, especially also radioactive nickel,
from the supernova core to the stellar envelope in hydrodynamical
explosion models. Measurements of neutrino and gravitational-wave
signals in the lucky case of a future galactic supernova are likely
to reveal correlated signal features that provide direct evidence
of violent hydrodynamic instabilities in the first second of the
explosion.
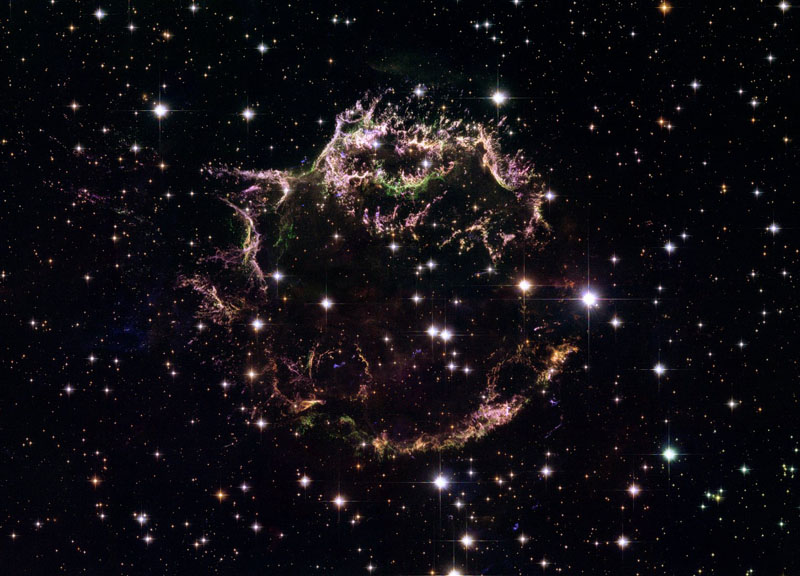
Figure 3a:
The Cassiopeia A supernova remnant in optical light. The image
was taken by NASA's Hubble Space Telescope. The picture reveals
a complicated and asymmetric structure of the expanding fragments
of a star that exploded only about 330 years ago.
(Credit: NASA/ESA/Hubble Heritage (STScI/AURA))
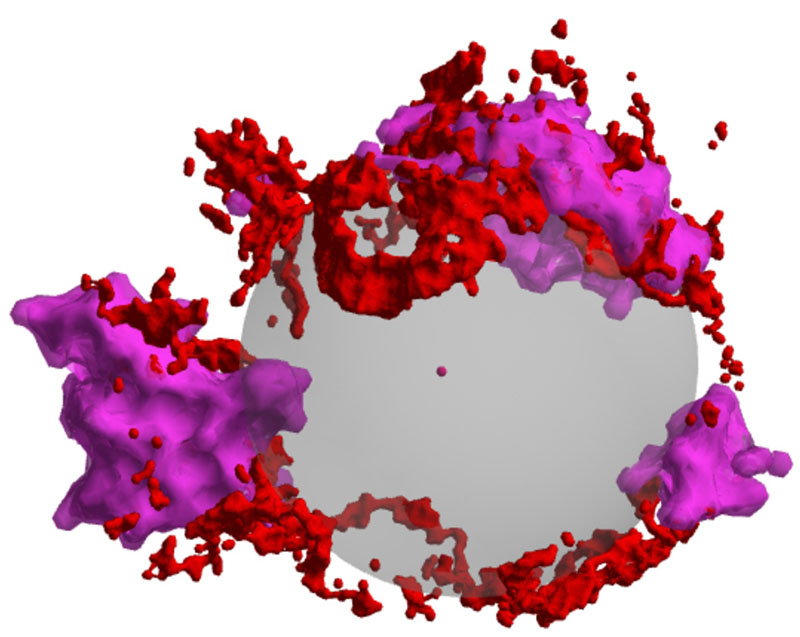
Figure 3b:
Visualization of different components of the ejecta of the
Cassiopeia A remnant. Red represents sulfur and oxygen whose
presence is deduced from optical radiation. Purple is X-ray
emitting iron. The point in the center is the geometrical
origin to which the expansion of the stellar fragments points
back. The translucent sphere is a visual aid.
(Courtesy of Robert Fesen and Dan Milisavljevic; using
iron data taken from DeLaney et al. (2010))
First-principle models of core-collapse supernovae heavily
rely on a reliable description of the microphysics that plays
a role in the supernova core and nascent neutron star. In particular
the equation of state of dense and hot matter and the neutrino
interaction rates in plasma at extreme conditions determine
the collapse and explosion dynamics and the nucleosynthesis
conditions in neutrino-heated ejecta. Observational constraints
like neutron star masses and radius estimates, as well as
theoretical and experimental studies of nuclear matter, begin
to set serious constraints on the properties of the high-density
equation of state. Considerable efforts are being made for developing
consistent new models that account for all of these constraints
and that can be supplemented by a consistent description of the
neutrino opacities. A new, forthcoming generation of supernova
models based on this improved input will yield more reliable
predictions of neutrino spectra and luminosities and may exhibit
interesting new twists of the role of neutrino flavor
transformations and neutrino-induced nucleosynthesis in supernovae.
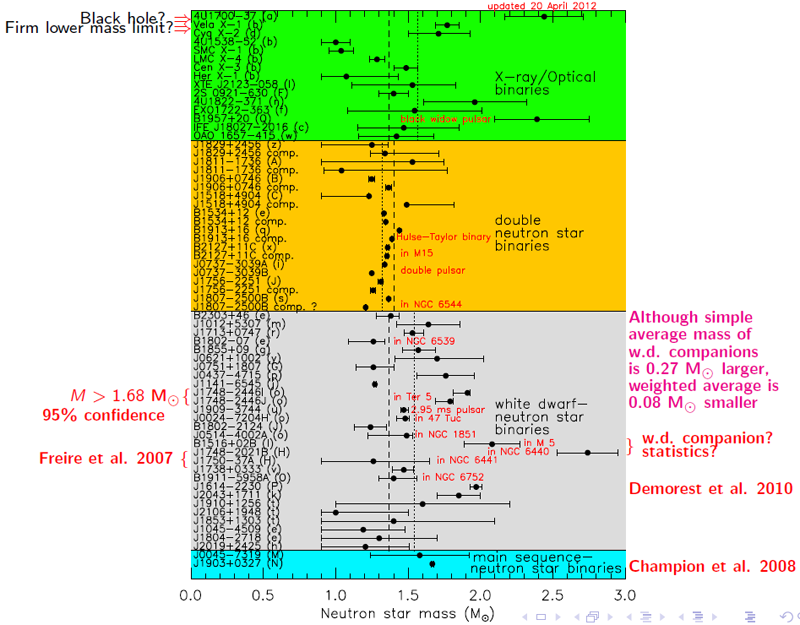
Figure 4:
Neutron star mass determinations and estimates for different
types of binary systems. In particular the 1.97 solar-mass pulsar
of Demorest et al. (2010) has ruled out a large variety of models
for "soft" neutron star matter. Only few of the currently available
nuclear equations of state for supernova simulations are compatible
with both the mass and radius constraints deduced from astrophysical
and experimental data. (Courtesy of James Lattimer)
|